Recently, the crystallization team at Porton and Genentech, Inc. jointly published a paper titled “Phase Transitions Involving Channel Hydrates of a New Pharmaceutical Compound” in 《Pharmaceutical Research》, the official journal of the American Association of Pharmaceutical Scientists. This journal focuses on publication of new research results in the combined area of medicine and chemistry.
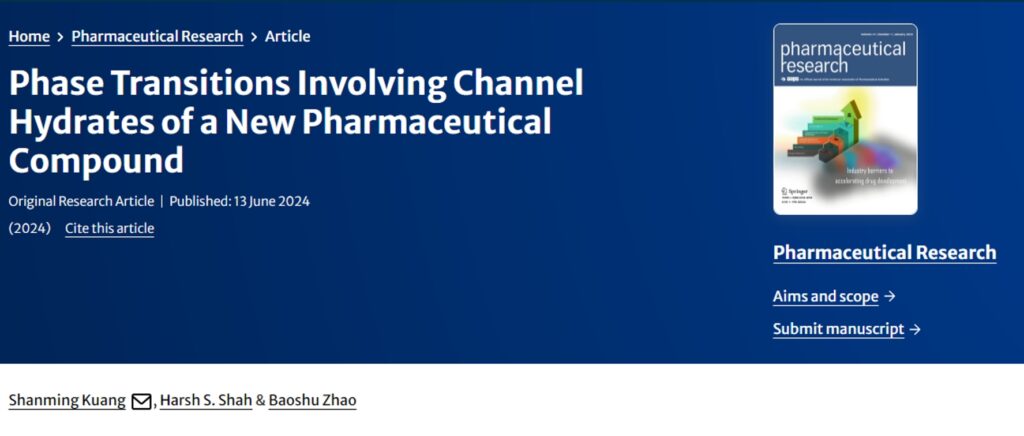
Polymorphism is one of the crucial activities during the drug discovery and development stage in the pharmaceutical industry. At the same time, hydrates are just another way to improve the physico-chemical properties of a pharmaceutical compound by modifying its crystal structures without having any toxicity concerns. However, the level of hydration in the hydrate can have a significant impact on physico-chemical properties, such as crystallinity, hygroscopicity, solubility, stability, processability, and dissolution rate. For these reasons, it is equally important to screen different hydrates of a pharmaceutical compound with possible structure determination to understand the mechanism of hydrate formation and dehydration kinetics to assess the potential risks involved during drug manufacturing processes.
This research investigation focuses on the different phase transition behaviors of a newly discovered pharmaceutical compound with three channel hydrates, two of which were confirmed by single-crystal analysis. Hydrate 1 was found to convert to Hydrate 2 in dry acetone as well as in dry THF. This suggests that Hydrate 2 is more stable under low water activity, which could further transform to Hydrate 3 when water activity increases. Hydrate 2 also transformed to Hydrate 3 in the presence of moisture. Follow-up experiments confirmed that Hydrate 2 converted to Hydrate 3 in water-containing solvents of high water activity, such as acetone/water. The transformation to Hydrate 3 was also achieved in acetone/water by starting with Hydrate 1 and the anhydrate form. Additionally, Hydrate 1 even transformed to Hydrate 3 in the solid state under ambient conditions. Additionally, Hydrate 1 even transformed to Hydrate 3 in a solid state under ambient conditions, indicating Hydrate 3 is more stable at high relative humidity.
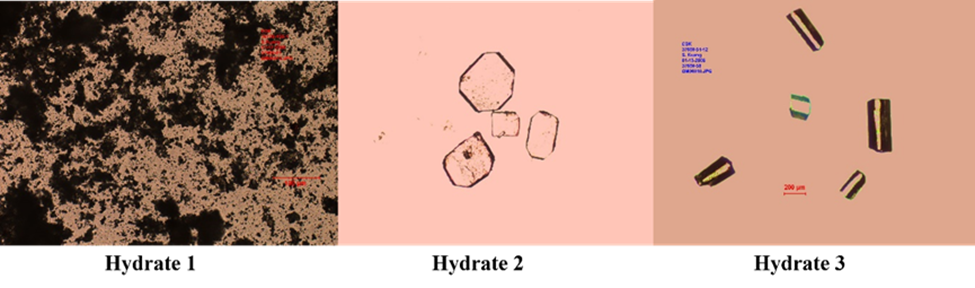
Fig. 1: Morphology of channel hydrates.
The dehydration/rehydration behaviors of Hydrates 2 and 3 were investigated using X-ray diffraction (XRD). No significant change in the PXRD pattern was observed when Hydrate 2 was stored under ambient conditions for 5 days, indicating no expansion or change in the crystal lattice (Figure 2). This suggests that the unit cell of Hydrate 2 is quite rigid and may not accommodate additional water without altering the crystal lattice. However, upon heating to 120 °C for 30 minutes, a change in the PXRD pattern of Hydrate 2 was observed, indicating potential transformation in crystal structure upon dehydration. The change in crystal structure was also illustrated by the collapse of a single crystal that was heated from room temperature (RT) to 120 °C. Interestingly, as shown in Figure 2, if the dehydrated form was cooled down to RT for 30 minutes under ambient conditions, the original PXRD pattern of Hydrate 2 was obtained, indicating reversible dehydration/rehydration processes, although a new crystalline phase was involved.
To further understand the reversible phenomenon in the crystal lattice, the crystal structure was packed to understand the presence of the water channel and its association with any given planes. It was apparent that the water molecules were lined up along the (202) and (-202) planes (highlighted in blue) in Hydrate 2, associated with the peak observed at 17 °2Ɵ. The peak intensity for this peak decreased significantly, and a new set of peaks, such as those at 11.7, 12.9, 13.7, 17.5, and 18.1 °2Ɵ, appeared upon dehydration, suggestive of a crystal structure with a different unit cell, as opposed to a lattice contraction/relaxation observation with normal channel hydrates. Interestingly, despite resulting in a totally different XRPD pattern upon dehydration, upon exposure to ambient humidity conditions (Figure 2), the dehydrated form converted back to the initial hydrate. This phenomenon for Hydrate 2 as a channel hydrate is unique and demonstrates the novelty of this work.
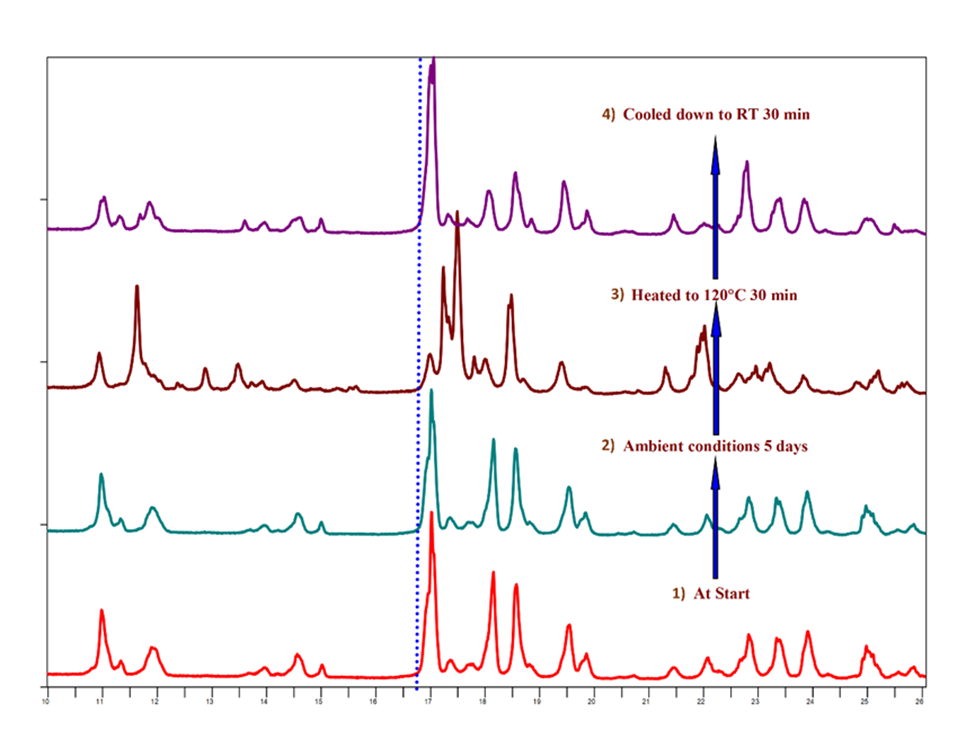
Fig.2:Dehydration/rehydration behavior of Hydrate 2.
Different from the behavior of Hydrate 2, the PXRD pattern of Hydrate 3 shifted to a lower angle when it was stored under ambient conditions for 5 days (Figure 3), confirming a unit cell expansion by absorbing moisture. The PXRD pattern shifted back to a higher angle when it was heated to 120°C for 30 minutes, revealing that water was driven out of the crystal lattice by heating. This is most likely a single crystal dehydration, as evidenced by the unaltered morphology of a single crystal that was heated from room temperature (RT) to 120°C. The retention of the overall morphology of the single crystal suggested a topotactic dehydration process. If the dehydrated form was cooled down to RT for 24 hours, its PXRD pattern once again shifted to a lower angle, indicating that it picked up moisture. Therefore, the dehydration/rehydration processes were reversible, and the crystal structure was retained upon dehydration, as evidenced by a similar PXRD pattern between the hydrated and dehydrated forms.
Similar to Hydrate 2, the planes (020) and (002) were identified as water channels in Hydrate 3, associated with the peak observed at 18.5° 2Ɵ. Upon heating Hydrate 3 to 120°C, this peak shifted to a higher angle at 19.0° 2Ɵ, clearly suggesting lattice contraction in the -x and -y directions. Another channel was observed along the (0-12) plane, associated with a peak at 11.0° 2Ɵ, which did not show any peak shift upon heating, suggesting this channel’s participation in crystal lattice stabilization. Under ambient conditions, channels along planes (020) and (002) allowed the re-entry of water molecules, potentially rearranging the crystal lattice to attain Hydrate 3.
The reversible behavior was further demonstrated by DVS, which also showed reversible absorption and desorption curves, indicating that the water molecules in Hydrate 3 can be mobilized easily and migrate in and out of the crystal lattice as a function of temperature and relative humidity.
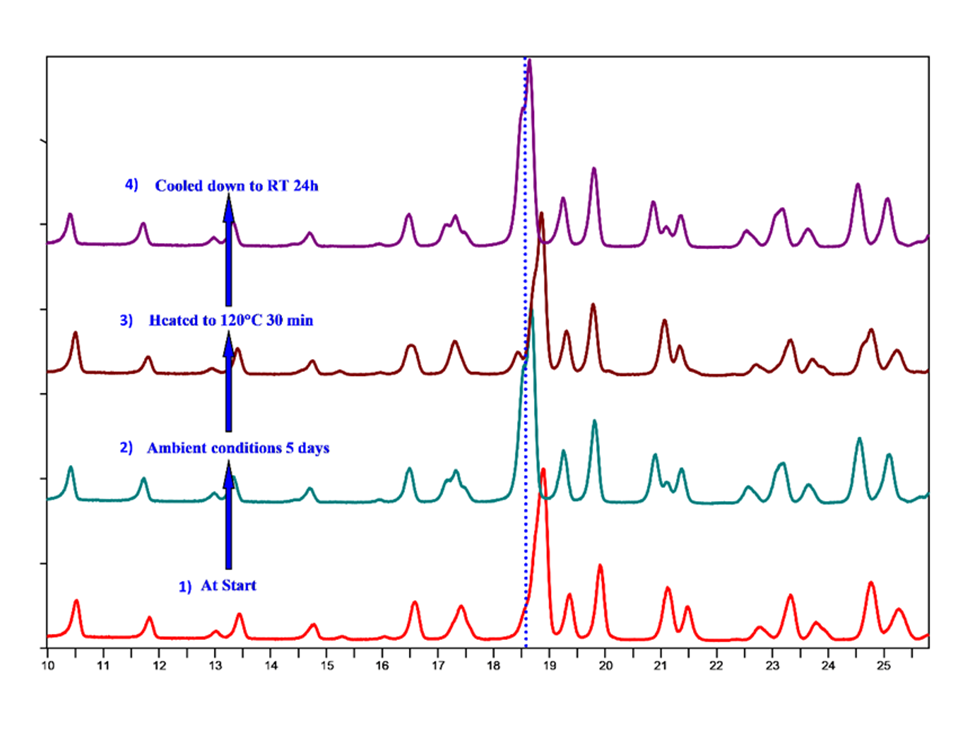
Fig.3:Dehydration/rehydration behavior of Hydrate 3.
As indicated by the data above, Hydrate 2 and Hydrate 3 exhibit different dehydration behaviors. As observed in Hydrate 2, the transition from the hydrate to the dehydrated form changed the long-range order structure, as indicated by the PXRD pattern change. However, it may have retained the short-range order structure because the dehydrated form converted back to hydrate form once exposed to moisture. It is expected that a channel hydrate will become a metastable form with high energy when water is driven out of the crystal lattice (Figure 4). For this high-energy metastable species, there are two primary pathways to stabilize the dehydrated lattice. One way is to absorb water and return to its original hydrate form, while the other is to undergo lattice relaxation at high temperatures, to reduced unit cell volume further resulting into increased crystal packing efficiency. If lattice relaxation is symmetric, a similar XRD pattern, except for peak changes, is expected, which is the case for Hydrate 3. On the other hand, a different XRD pattern peak changes is expected, which is the case of Hydrate 3. On the other hand, a different XRD pattern is generated in Hydrate 2 suggesting the lattice relaxation is non-symmetric at high temperatures.
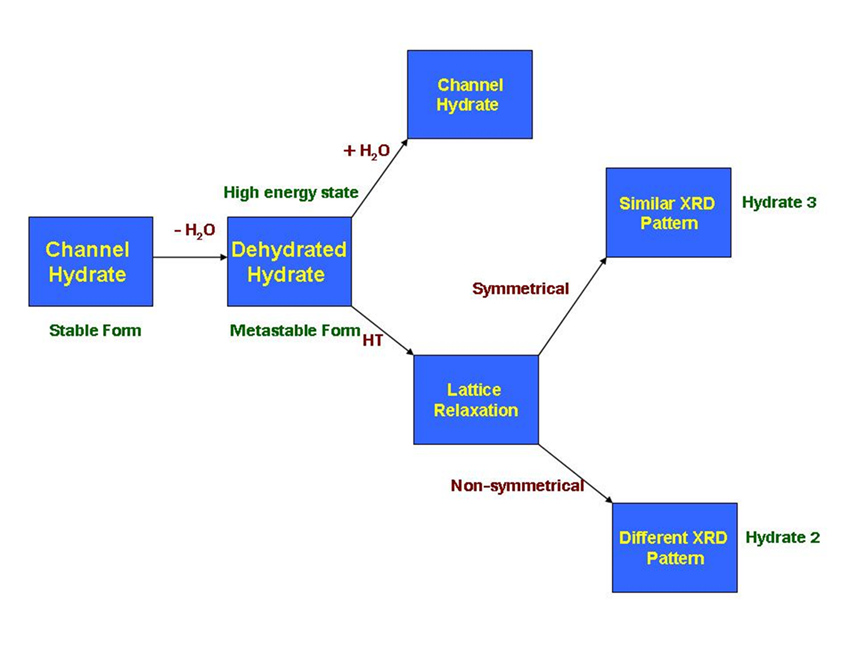
Fig.4: Crystal lattice change upon dehydration of Hydrate 2 and Hydrate 3.
In conclusion, this paper explores the critical role of hydrates as active pharmaceutical ingredients (APIs) using advanced analytical techniques. We discovered unique phase transition behaviors in a new pharmaceutical compound with three channel hydrates, revealing both symmetric and asymmetric lattice relaxations. These findings are crucial for ensuring hydrate stability in drug manufacturing and storage, providing valuable insights for future pharmaceutical developments.
The crystallization team at Porton, with locations in New Jersey (USA) and Shanghai (China), offers expert services in polymorph discovery and selection, crystallization process development, particle engineering, DS-DP co-processing, and pre-formulation evaluation, and has played a key role in advancing drug development programs globally. Collaborating with global partners, the team is dedicated to addressing challenges presented by different forms and supporting a smooth transition through each phase of development. The team will continue exploring new frontiers in small molecule pharmaceuticals and innovative modalities, providing top-notch services from preclinical to commercial phases.
Phase Transitions Involving Channel Hydrates of a New Pharmaceutical Compound. Shanming Kuang, Harsh S. Shah, Baoshu Zhao. Pharmaceutical Research,